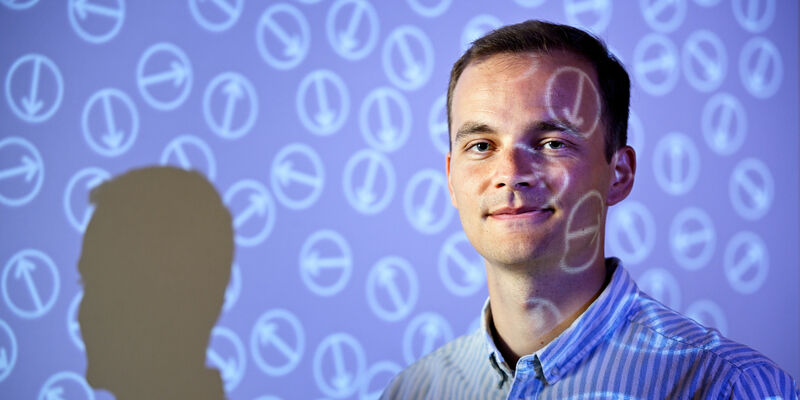
- Research , Home Stretch
- 06/07/2023
Home Stretch | Mobile cancer cells modeled
Why do tumor cells stay put under certain conditions, but become mobile in others, raising the prospect of metastasis? TU/e researcher Vincent Debets looked at the cells in the human body from a physics perspective and developed a unique model designed to boost our understanding of complex cell movements. On Friday July 7th he defends his research at the Department of Applied Physics and Science Education.
Peering at his computer screen, Vincent Debets points out the minuscule movement of a large group of tiny spheres. These spheres and the theory of active matter led him to develop a model designed to better predict the behavior of various entities, among them cancer cells. Debets has spoken about his work on a previous occasion, for the BNR podcast Science Today. Some scientists study nice neat cells with protrusions and all sorts of organelles, but not him. He narrowed his focus down to a simple sphere. With computing power opening up new avenues of inquiry, biological processes are among the new fields in the spotlight, explains Debets.
“Biophysics is burgeoning. How can you apply the principles of physics to tackling a complex biological problem and can this approach then be built into a model used to make calculations? We are looking at complex cellular behavior from a different perspective. Biologists are attempting to map everything they can observe. But in many cellular processes there's so much happening simultaneously that it's difficult to establish the driving force behind what you're seeing. And so we are now taking the first steps towards using a physical model to describe biological cellular behavior.”
Irregular cells
In order to study a cell's movement in a systematic way, Debets delved into the world of glass. Whereas you and I might think of glass as a window, vase or beer glass, in physics 'glass' is a much broader concept, Debets explains patiently. “In a physical sense glass is associated with one of the three forms materials can take, known as the three phases of matter: gas, liquid and solid. In a liquid, molecules can move about freely; in the solid phase molecules are fixed in an orderly crystal lattice. But if you cool or compress matter quickly enough, the molecules don't have the opportunity to tidily arrange themselves. What results instead is an intermediate form, a solid with an irregular structure: the glass phase.
Cells too can behave like glassy material. In, a tumor, for example, cells don't organize into a regular structure. And in some circumstances cancer cells stay put, while in others they are able to move through another cellular layer, as in the liquid state. So in physical terms, something akin to a glass transition appears to be occurring.”
Predictable behavior
Debets decided to regard cells as entities, perfect spheres. But, he is keen to emphasize, there is a crucial difference between these spheres and the molecular spheres in a crystal lattice. “Cells are active. They have internal combustion engines and can independently convert energy into motion. Molecules cannot do this. In a tumor we see ‘fluid’ domains with a great deal of motion and domains where cells stay put. How does activity change a cell's behavior? What causes cells to detach from the tumor, giving rise to the possibility of metastasis? And are we talking about discrete cells or a cell cluster? We want to predict in the simplest way possible what a cancer cell is going to do. The activity keeps the system in equilibrium, something we also see in active glassy matter. I hunted for a usable model in the field of glass theory, then tried to add to that the element of activity. You start out very simply, modeling a material as it transitions to a dense, irregular structure. How do spheres move in this material? And what happens when I start varying the properties of the activity, how does that change the dynamics?”
Hard spheres
While earlier research findings were not unanimous, Debets's unique model was able to explain the differences. He found that where the description of the degree of ‘fluid’ motion was concerned, the parameter ‘length’ was much more important that the parameter ‘time’. “We now have a good understanding of the simplest model. For the first time, we are seeing universal behavior and can predict which reaction a particular activity will elicit.”
Time then to add another layer of complexity. Whereas in his earlier simulations Debets used hard spheres, in his subsequent studies he made them softer. “A first step towards a more cell-like, deformable context. Now the spheres show a little springiness when they come together, their interactions are more gentle. But there's no loss of that universal behavior we identified. We're in a position now to systematically add or exclude things; which elements are important factors in determining a cell's movement behavior?”
Experimental feedback
Naturally, a question mark hangs over the extent to which a relatively simple model can be used to describe complex cell behavior, as Debets realizes all too well. And so he advocates working more closely with ‘experimentalists’. “The biggest challenge lies in establishing which parameters need to be included in your model. Then, by way of experimental feedback – which biological factors are actually important – we can adapt our models in order to create the most realistic setting possible. And then we can significantly broaden our focus, a big advantage of simulations. After all, in biological experiments it's much harder to control the variables.”
As a recent example of the importance of having a strong experimental link, Debets cites the finding that the cell nucleus plays a very important role. Many models now in use regard the cell as a homogeneous entity, but the nucleus is much harder than the watery cytoplasm surrounding it. This means that if you have a dense packed cell layer, it's all the more important that the hard cell nucleus finds somewhere it can maneuver its way through. How do you include this element in your model? Debets: “Issues like these require multiple perspectives; within our discipline we are seeing two different worlds being brought together. Gradually, we are seeing more and more links between physicists, biologists, chemists; and this is essential. Here at TU/e we're also fostering this approach, for example the ICMS research institute is part of a consortium with eminent cancer biologists. To an outsider, it may seem impossible to capture human cells in a physical model, but we really are conducting the research of the future here.”
Discussion